Future Prospects of Microfluidic Chip Applications in the In Vitro Diagnostics Field
Share this Post to earn Money ( Upto ₹100 per 1000 Views )
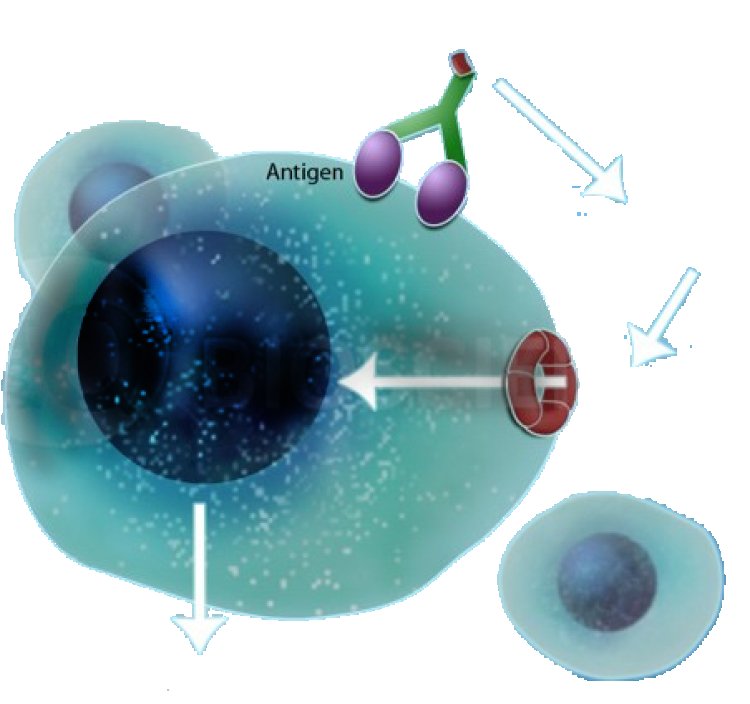
The global market for microfluidic products surged to $9.98 billion in 2019, with microfluidic devices accounting for $3.48 billion of this figure. A notable trend in the industry is the ongoing acquisition of microfluidic companies by larger enterprises, signaling a trajectory of accelerated growth through capital infusion.
In the industrial landscape, in vitro diagnostics (IVD) stands out as the primary sector for microfluidic applications, driven by its lucrative returns. Demographic shifts, particularly aging populations, contribute to an escalating demand for microfluidic chips. Moreover, governmental policies prioritize the advancement of the microfluidics industry, a focus that has intensified amidst the backdrop of the pandemic. Moving forward, the critical hurdles facing microfluidic chip technology revolve around manufacturing costs and scalability. Achieving scalable production processes and cost reduction measures while maintaining product standardization and minimizing variations are imperative objectives.
The evolution of modern technology emphasizes miniaturization, integration, and intelligence. Microelectromechanical systems (MEMS) have played a pivotal role in this evolution, enabling the transition from bulky electronic systems to compact integrated circuit chips and handheld devices like smartphones. Similarly, microfluidic chips, often referred to as Lab-on-a-Chip technology, epitomize the manipulation of fluids at micro- and nanoscales. These chips condense essential laboratory functionalities, such as sample preparation, reaction, separation, and detection, onto a compact chip, typically a few square centimeters in size. The hallmark of microfluidic chips lies in their capacity for flexible integration and scaling of diverse unit technologies within a controllable microplatform.
Originating from MEMS technology, early microfluidic chips underwent fabrication processes on substrates like silicon, metals, polymers, glass, and quartz. These processes yielded microstructure units such as fluid channels, reaction chambers, filters, and sensors, with dimensions ranging from micrometers to sub-millimeters. Subsequent fluid manipulation within these microstructures enabled automated execution of biological laboratory procedures, including extraction, amplification, labeling, separation, and analysis, or cell manipulation and analysis.
In the early 1990s, A. Manz et al. demonstrated the potential of microfluidic chips as analytical chemistry tools by achieving electrophoretic separation—a technique previously confined to capillaries—on chips. Subsequently, spurred by the U.S. Department of Defense's requisition for portable biochemical self-test equipment, research in microfluidic chips burgeoned globally. Throughout the 1990s, microfluidic chips primarily served as platforms for analytical chemistry, often interchangeably referred to as "Micro Total Analysis Systems" (u-TAS). Consequently, these chips found applications across diverse fields, including biomedical diagnostics, food safety, environmental monitoring, forensics, military, and aerospace sciences.
Key milestones in the advancement of microfluidic chips include G. Whitesides et al.'s 2000 publication on PDMS soft lithography and S. Quake et al.'s 2002 article on "large-scale integration of microfluidic chips" featuring microvalve and micropump controls. These seminal works propelled microfluidic chips beyond the confines of traditional analytical systems, unlocking their potential for significant scientific and industrial applications. For instance, microfluidic chips enable the execution of combinatorial chemical reactions or droplet techniques, facilitating drug synthesis, high-throughput screening, and large-scale nanoparticle or microsphere production. In essence, microfluidic chips pave the way for the realization of a "chemical plant or pharmaceutical lab on a chip."